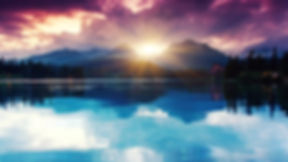
Life & Observation
De-icing Externalities
This week, the focus of this blog is another negative externality usually overlooked: de-icing. Although residents in my hometown (Hangzhou, just about 30 degrees north in latitude) do not see de-icing as a routine winter sight, I have experienced life in places where de-icing is a must for three months or more.
In Corner Brook, Newfoundland, icing conditions on road can literally prevent any vehicles and even pedestrians from attempting to use the road at all, for ice-covered hilly paved roads can be effectively non-usable. Probably due to this necessity, de-icing salt pollution is usually overlooked in many regions, or regarded as the “necessary evil” to keep daily life running.
Out of maintenance purposes, workers and the academia have long admitted the corrosive nature of many kinds of de-icing chemicals used, for they do corrode drainage infrastructure and wastewater treatment facilities (Vítězová et al., 2016). Yet in fact, studies have more than once proved that not only would de-icing salt flow off the road with melting water and enter the ecosystem, the high salt concentration of such melting water can indeed damage biodiversity of local flora, if not fauna as well (Li et al., 2014). On more specific grounds, because melting water is rarely completely cleaned by wastewater treatment facilities, melting salt commonly becomes part of surface runoff and joins natural watersheds. If not correctly balanced, high salinity pollutant can (1) join groundwater system and impact water quality, while also adding to the risk of soil salinization (Espinha Marques et al., 2019); (2) directly impact off-road greenspace, especially in rural areas where pavement runoff can immediately join adjacent ecosystem (Norrström & Bergstedt, 2001); (3) in long term affect chemical balance of major water bodies upon which both human and ecosystem rely.
Solutions regarding this kind of externalities do exist. Except from chemical de-icing involving salt, light icing on pavement surface can also be neutralized with gravel of suitable size and shape that provides necessary friction. The most common treatment procedure of de-icing water involves guiding saltwater along an artificial channel to designated collection point, where saltwater can then be correctly balanced. novel methodologies aiming to balance de-icing salt with microorganisms have also been developed (Guesdon et al., 2016). Unlike previously discussed externalities, the main setback regarding the clearing of such externality is whether authorities have necessary will to put measures in place.
References
Espinha Marques, J., Marques, J. M., Carvalho, A., Carreira, P. M., Moura, R., & Mansilha, C. (2019). Groundwater resources in a Mediterranean mountainous region: environmental impact of road de-icing. Sustainable Water Resources Management, 5(1), 305–317. https://doi.org/10.1007/s40899-017-0170-z
Guesdon, G., de Santiago-Martín, A., & Galvez-Cloutier, R. (2016). Phytodesalinization potential of Typha angustifolia, Juncus maritimus, and Eleocharis palustris for removal of de-icing salts from runoff water. Environmental Science and Pollution Research, 23(19), 19634–19644. https://doi.org/10.1007/s11356-016-7176-1
Li, Z., Liang, Y., Zhou, J., & Sun, X. (2014). Impacts of de-icing salt pollution on urban road greenspace: a case study of Beijing. Frontiers of Environmental Science & Engineering, 8(5), 747–756. https://doi.org/10.1007/s11783-014-0644-2
Norrström, A.-C., & Bergstedt, E. (2001). The Impact of Road De-Icing Salts (NaCl) on Colloid Dispersion and Base Cation Pools in Roadside Soils. Water, Air, and Soil Pollution, 127(1), 281–299. https://doi.org/10.1023/A:1005221314856
Vítězová, M., Vítěz, T., & Nitayapat, N. (2016). Effects of De-icing Salts on the Respiration of the Microorganisms of Activated Sludge. Water, Air, & Soil Pollution, 227(11), 416. https://doi.org/10.1007/s11270-016-3119-1